Photovoltaic systems convert sunlight directly into electricity. The residential photovoltaic system can meet some or all of the daily electricity demand of the household in the form of a photovoltaic roof. The photovoltaic system can also be equipped with a backup battery, which can continue to supply power to the load when the power grid is out of control.
This manual mainly proposes the design and installation solutions for household grid-connected photovoltaic systems. It provides installers with methods and guidelines on choosing photovoltaic products, helping them accurately install household photovoltaic power generation systems to make the design system Unleash your potential.
Ⅰ. Basic steps to follow to install a rooftop photovoltaic system
(1). Make sure the roof or other installation location is sized to accommodate the PV system that will be installed.
(2). During installation, it is necessary to check whether the roof can withstand the quality of the other photovoltaic system. If necessary, it is necessary to enhance the bearing capacity of the roof.
(3). Properly handle the roof according to the design standards of the building roof.
(4). Install the equipment strictly by the specifications and procedures.
(5). Correct and well-set grounding system can effectively avoid lightning strikes.
(6). Check if the system is running well.
(7). Ensure that the design and associated equipment can meet the grid connection needs of the local grid. 8. Finally, the system is thoroughly tested by traditional testing agencies or power departments.
Ⅱ. Problems related to system design
Types of photovoltaic power generation systems: one is a photovoltaic power generation system that is connected in parallel with the public power grid and has no backup battery for energy storage; the other is a photovoltaic power generation system that is connected in parallel with the public power grid and also has a backup battery as a supplement.
(1). Grid-connected system without battery
Such systems can only operate when the grid is available. Because the power loss of the grid is minimal, such a system can generally save the user more electricity bills. However, in a power outage, the system will shut down entirely until the grid is restored, as shown in Figure 1.
A typical battery-free grid-connected system consists of the following components:
1) Photovoltaic array.
Photovoltaic arrays consist of photovoltaic modules, which are made up of solar cells connected in some way and sealed. Usually, a collection consists of several photovoltaic modules connected by brackets.
2) Equipped with balance system (BOS)
It is used for bracket systems and wiring systems, including integrating photovoltaic modules into home building systems' electrical systems. The power supply line system includes:
3) DC-AC inverter
This device converts direct current from photovoltaic arrays into the standard alternating current used by household appliances.
4) Measuring instruments and meters
These instruments measure and display system operating status, performance, and user power usage. 5) Other components
Utility grid switch (this depends on the local utility grid).
(2). Grid-connected system with battery
This kind of system adds batteries to the grid-connected system without batteries to store energy for the system. Even in a power outage, the system can provide an emergency power supply for special loads. When the power is interrupted, the system is separated from the grid to form an independent power supply line. A dedicated distribution line is used to supply power to these special loads. If the power failure of the grid occurs during the day, the photovoltaic array can supply power to these loads together with the battery; if the power failure occurs at night, the battery will supply power to the load, and the battery can release enough energy to ensure the regular operation of these special loads.
In addition to all the components in a grid-connected system without a battery, a battery backup system also needs to add batteries and battery packs, battery charge controllers, and switchboards that supply power for loads with special requirements and high security.
Ⅲ. Installation of rooftop photovoltaic system
1). roof structure
The most convenient and appropriate place to install a photovoltaic array is on the roof of a building. For pitched roofs, the photovoltaic array should be installed on the roof parallel to the roof surface, with brackets separated by a few centimeters for cooling purposes. If it is a horizontal roof, it is also possible to design a bracket structure that optimizes the inclination angle and install it on the top. The roof-mounted photovoltaic system must pay attention to the sealing of the roof structure and the roof anti-permeability layer. Generally, one support bracket is required for every 100 watts of PV modules. For a new building, the support brackets are usually installed after the roof decking has been installed and before roof waterproofing has been installed. The staff responsible for the array mounting system can install the support brackets while installing the roof.
Tile roofs are often structurally designed to close their load-bearing capacity limits. In this case, the roof structure must be reinforced to withstand the additional weight of the PV system, or the tiled roof must be changed into a dedicated strip area to install PV arrays. However, if a tiled roof is converted to a lighter roofing product, there is no need to strengthen the roof structure because the combined mass of such a roof and photovoltaic array is lighter than the mass of the replaced tiled roofing product.
2). shade structure
An alternative to roof installations is a shading structure-mounted photovoltaic system. This shading structure maybe a patio or a double-layered shading grid, where the photovoltaic array becomes the shade. These shading systems can support small or large photovoltaic systems.
Such buildings with photovoltaic systems cost slightly differently than standard patio coverings, primarily when the photovoltaic array acts as a partial or complete shade roof. If the PV array is installed at a steeper angle than a typical shading structure, the roof structure will need to be modified to accommodate wind loads. The mass of the photovoltaic array is 15-25 kg/m², which is within the load-bearing limit of the shade support structure. The labor costs associated with installing roof brackets can be factored into the entire patio cover construction cost. The overall construction cost is likely to be higher than installing it on the roof, but the value generated by the shading structure often offsets those extra costs.
Other issues to consider include: simplifying the maintenance of the array, the wiring of the components, the connection of the wires must remain aesthetically pleasing, and creeping plants must not be grown or pruned to keep the members and their wiring undisturbed.
3). Building Integrated Photovoltaic (BIPV)
Another type of system replaces some traditional roofing products with building-integrated photovoltaic arrays. When installing and using such products, care must be taken to ensure that they are installed correctly, achieve the necessary fire rating, and require proper installation to avoid roof leaks.
Ⅳ.estimate the system output
1). Standard Test Conditions
Solar cell modules generate direct current. The manufacturer calibrates the DC output of the solar module under standard test conditions. While these conditions are easily achieved in the factory and allow products to differ from one another, these data need to be corrected to evaluate their output power when operating in outdoor conditions. The standard test conditions are a solar cell temperature of 25°C, a solar radiation intensity of 1000 watts/square meter (commonly referred to as peak sunlight intensity, which is equivalent to the radiation intensity at noon on a clear summer day), and a mass of 1.5 AM when passing through the atmosphere. Filtered solar spectrum (ASTM standard spectrum). Manufacturers refer to solar modules with an output of 100 watts as measured under standard test conditions as "100-watt solar modules". The rated power of this battery pack is allowed to deviate from the actual value by 4-5%. This means that a 95-watt module is still called a "100-watt module". A lower output power value should be used as a basis (95 watts instead of 100 watts).
2). temperature effect
The module's output power decreases as the module's temperature increases. For example, when the sun shines directly on the photovoltaic roof module, the internal temperature of the module will reach 50℃~75℃. For monocrystalline silicon modules, the increase in temperature will cause the module power to drop to 89% of the actual power. Therefore, a 100-watt module can only produce about 85 watts (95 watts x 0.89 = 85 watts) when it is hit by full sunlight at noon in the spring or fall.
3). Dirt and Dust Effects
The accumulation of dirt and dust on the surface of the solar panel will affect the transmission of sunlight and reduce the output power. Most areas have rainy and dry seasons. Although rainwater can effectively clean the dirt and dust on the surface of the module during the rainy season, a more complete and adequate estimation of the system should consider the power reduction caused by the dirt on the surface of the panel during the dry season. Due to dust factors, the system power is generally reduced to 93% of the original rated value every year. So this "100-watt module" runs at an average power of 79 watts (85 watts X 0.93 = 79 watts) with dust on the surface.
4). Matching and Line Loss
The maximum power output by the overall PV array is generally less than the sum of the total power output by individual PV modules. This discrepancy is caused by inconsistencies in solar PV modules, also known as module misalignment, which will cause the system to lose at least 2% of its electrical energy. In addition, electrical power will also be lost in the internal resistance of the line system, this part of the loss should be kept to a minimum. Still, it is difficult to reduce this part of the loss to the system when the power peaks at noon, and then in the afternoon Gradually decreasing again; the power will return to zero value at night; this change is attributed to the evolution of solar radiation intensity and the development of the sun angle (relative to the solar cell module). Furthermore, the inclination and orientation of the roof will affect the grade of sunlight hitting the surface of the module. The specific manifestations of these effects are shown in Table 1, indicating that if the local photovoltaic array is placed on the roof with a slope of 7:12, the correction factor facing due south is 100, when the slope angle of the roof is less than 3% of the energy. Therefore, a reasonable loss factor should be 5%.
5). DC to AC Conversion Losses
The DC power generated by the solar modules must be converted into standard AC power by an inverter. Some energy will be lost in this conversion process, and some points will be lost in the wiring from the rooftop components to the inverter and the customer switchboard. At present, the peak efficiency of inverters used in home photovoltaic power generation systems is 92% to 94%, which is the peak efficiency given by inverter manufacturers and is measured under good factory control conditions. In fact, under normal circumstances, the efficiency of the DC-AC inverter is 88%~92%, and 90% is usually used as a reasonable compromise efficiency.
Therefore, a "100-watt module" with reduced output due to product deviation, heat, wiring, AC inverter, and other power losses, at noon with a clear sky, only a maximum of 68 watts of AC power is delivered to the user's switchboard. (100WX095×0.89×0.93×095X0.90—68W).
6). Influence of sun direction angle and house orientation on system energy output
Throughout the day, the angle at which the sun's rays strike the solar panel is constantly changing, which will affect the output power. The "100-watt module" output power will gradually increase from the zero value at dawn, with the change of the sun bearing angle, the same degree. Still, the array is facing east; the power produced will be 84% of the power facing south (corrected in Table 1 factor of 0.84).
Ⅴ.System installation
1. Recommended materials
•Materials used outdoors should be resistant to sunlight and UV rays.
•Polyurethane sealants should be used on non-flash roof waterproofing. 3) Materials should be designed to withstand the temperature when exposed to the sun.
•Different metal (such as iron and aluminum) materials should be isolated from each other with insulating spacers, washers, or other methods.
•Aluminum should not be in direct contact with some materials.
•High-quality fasteners should be used (stainless steel is preferred).
•Structural member materials can also be selected: aluminum profiles, hot-dip galvanized steel, coated or painted ordinary carbon steel (only used in low-corrosion environments), stainless steel.
2. Recommended equipment and installation method
1)Make a list of all electrical equipment according to the rated voltage and rated current required in the application.
2) List the PV modules according to the relevant standards, and make sure that it has a shelf life of at least five years (20 to 25 years of life).
3) List the inverter according to the relevant standard, and make sure that it has a service life of at least five years. 4) Exposed cables and pipes should be resistant to light.
5) The system should have overcurrent protection and easy maintenance.
6) Terminals related to electricity should be tightened and fastened.
7) The manufacturer's installation instructions should install the equipment.
8) All roofs should be sealed with an approved sealant.
9) All cables, pipes, exposed conductors, and wire boxes should comply with relevant standards and regulations and ensure safety.
10) It should be ensured that the photovoltaic array is not shaded from 9:00 to 16:00 every day.
3. Matters needing attention in photovoltaic system design and installation
1) Carefully check the installation site of the photovoltaic array (such as roof, platform, and other buildings).
2) To ensure that the selected equipment is within the scope of local incentive policies.
3) Contact the local utility grid department to obtain grid connection and online test permission.
4) If it is installed on the roof when determining the installation position of the photovoltaic modules on the top, the influence of the building rainwater drainage pipes, chimneys and ventilation openings on the photovoltaic modules should be considered. Try to lay photovoltaic modules according to the size and shape of the roof to make the top more beautiful.
5) Calculate the sunlight exposure and shading of the installed photovoltaic array. If the chosen installation site has too much shade, you should consider changing where the PV array is installed.
6) Measure the distance between all system components, and draw the location diagram and schematic diagram of the photovoltaic system installation.
7) Collect relevant materials for relevant review departments, which should include the following:
①The location map shall show the location of the main system components - photovoltaic modules, pipeline wiring, electrical boxes, inverters, high-assurance load switchboards, utility grid on-off switches, main switchboards, and the inlet side of the utility grid.
②The schematic diagram should show all the essential electrical system components, as shown below picture
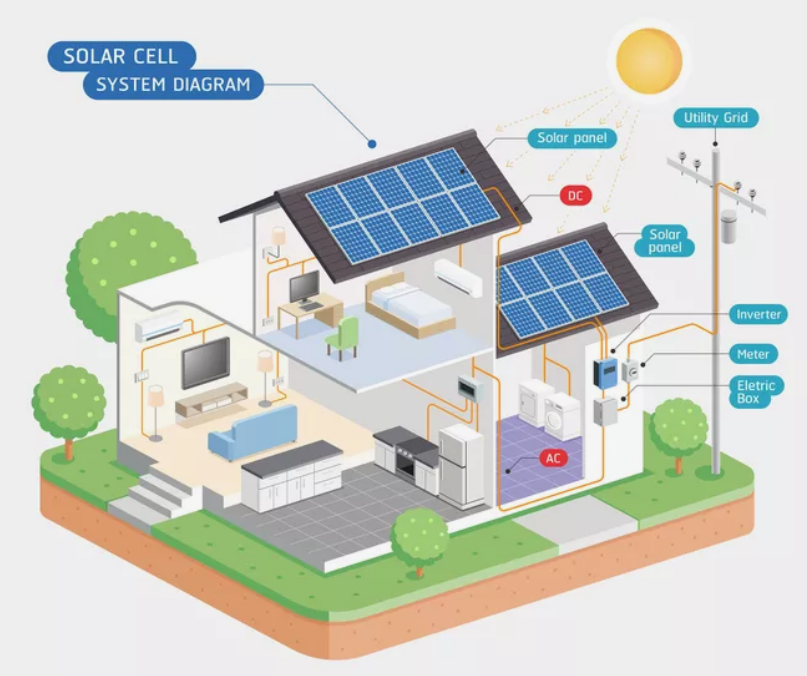
③Break down all critical electrical system components into small parts (photovoltaic modules, inverters, combiner boxes, DC switches, fuses, etc.).
8) Estimate the cable length from PV modules to combiner box and inverter
9) Check the current-carrying capacity of the photovoltaic module circuit, and determine the cable size suitable for the slightest current. The size of the cable is determined according to the maximum short-circuit current of each course and the length of the cable routing.
10) Calculate the size of the PV array, taking into account that at full power, the voltage drop from the PV module to the inverter is less than 3%. If the combiner box of the array is far from the inverter, then the voltage drop is not calculated based on the wiring from the PV array to the combiner box and the wiring from the combiner box inverter.
11) Estimate the line length from the inverter to the main switchboard.
12) Check the main switchboard to determine whether the power of the switchboard can meet the switching needs of the photovoltaic system.
13) If the system includes switchboards for support loads (with backup battery systems), identify specific critical load circuits.
These circuits should meet the expected electrical loads:
①Estimate the load connected to the backup system to meet the needs of actual power consumption and daily power consumption in the sleep state of the system.
② All backup loads must be connected to a separate switchboard for connection to the output of the dedicated inverter.
③The average power consumed by the load of the backup power system should be calculated to determine how long the energy storage in the battery can continue to supply power to the consumer.
④It is recommended to use a maintenance-free valve-regulated lead-acid battery system with adsorbed fiberglass wool because this battery does not require user maintenance.
⑤The battery's storage should avoid sunlight and be placed in a calm and ventilated place as much as possible. Whether it is a lead-acid solution or a valve-regulated lead-acid battery, it needs to be ventilated to the outside world.
14) Follow the design requirements
Cables connect PV modules, combiner boxes, overcurrent protectors/disconnect switches, inverters, and utility disconnect switches and ultimately join the circuit to the utility grid.
15) During the trial operation, the photovoltaic system circuit usually works, and the grid connection permit from the public power grid department is obtained. Then, the system can start to operate formally.
16) Observe whether the system instrument is running normally.
4. Maintenance and Operation Phase
1) When dust accumulates on the photovoltaic modules, the photovoltaic modules can be cleaned in cool weather.
2) Regularly check the photovoltaic system to ensure that the lines and brackets are in good condition.
3) Every year around March 21 and September 21, when the sun is full and close to noon, check the output of the system (the surface of the components is kept clean), and compare whether the operation of the system is close to the reading of the previous year. Keep this data in logs to analyze whether the system is always functioning correctly. If the readings drop significantly, there is a problem with the system.
Ⅵ. Inspection content and procedures of solar photovoltaic power generation system (it is recommended to wear safety helmet, gloves, and eye protection equipment)
1. PV array
1)Verify that all combiner box fuses are removed, and check that there is no voltage present at the output terminals of the combiner box.
2) Visually inspect whether any sockets and connectors between the PV modules and the switchboard are in normal working condition.
3) Check whether the stress-free clamp of the cable is installed correctly and firmly.
4) Visually inspect whether all PV modules are intact.
5) Check whether all cables are neat and fixed.
2. Circuit wiring of photovoltaic modules
1) Check the DC string combiner box (from the PV modules to the combiner box).
2) Recheck whether the fuse is taken out and all switches are disconnected.
3) Check whether the indoor cable lines are connected to the terminals of the DC series combiner box in the correct order, and make sure that the labels are visible.
3. Trace inspection of circuit string wiring
The following procedure is followed for each source circuit series in the system path (e.g., from east to west or north to south), with ideal test conditions being clear noon from March to October.
1) Check the open-circuit voltage of each component in the circuit to verify the actual voltage provided by the manufacturer on a sunny day (under the same sunlight conditions, there should be the same voltage. Note: under sunlight conditions, the have voltages above 20 volts).
2) Make sure that permanent cable markers can identify the positive and negative connections.
3) Check each component as above.
4. Other parts of photovoltaic array circuit wiring
1) Recheck that the DC disconnect switch is on and the labels are intact.
2) Verify the polarity of each branch power supply in the DC combiner box. According to the number of circuit strings and the position on the drawing, verify that the open-circuit voltage of each branch is within the appropriate range (if the sunlight irradiance does not change, the voltage should be very close).
Warning: If the polarity of any set of source circuits is reversed, this will cause a severe accident or even fire in the fuse unit, resulting in damage to the combiner box and adjacent equipment. The reversed polarity of the inverter will also cause damage to the system equipment, which is not covered by the equipment warranty.
3) Tighten all the terminals in the DC string combiner box.
4) Check that the neutral wire is connected correctly to the main switchboard.
5. Inverter startup test
1) Check the open-circuit voltage sent to the inverter DC disconnect switch to ensure that the voltage limits in the manufacturer's installation manual are met.
2) If there are multiple DC disconnect switches in the system, check the voltage at each switch.
3) Turn the power supply switch from the PV array to the inverter.
4) Confirm that the inverter is running, record the voltage of the inverter over time during operation, and confirm that the voltage reading is within limits allowed by the manufacturer's installation manual.
5) Confirm that the inverter can achieve the expected power output. 6) Provide a startup test report.
6. system acceptance test
Ideal PV system test conditions, choose sunny noonday from March to October. If ideal test conditions are not possible, this test can also be done at noon during a sunny winter day.
1) Check to ensure that the PV array is fully sunlit and without any shade.
2) If the system is not running, turn on the system running switch and let it run for 15 minutes before starting the system performance test.
3) Perform a solar irradiance test with one or two methods, and record the test value. Divide the highest radiation value by 1000 watts/square meter, and the data obtained is the radiation ratio. For example: 692w/m2÷1000w/m=0.692 or 69.2%.
Method 1: Test with a standard pyranometer or pyranometer.
Method 2: Find a normal operating photovoltaic module of the same model as the photovoltaic array, keep the same direction and angle as the photovoltaic array to be tested, and place it in the sun. After 15 minutes of exposure, use a digital multimeter to test the short-circuit current, and set the These values are recorded (in amps). Divide these values by the short-circuit current value (Isc) printed on the back of the PV module, multiply by 1000 watts/square meter, and record the results in the same row. For example: LSC measurement=36A; LSC printed on the back of PV module: 5.2A; actual radiation value=3.652A×1000w/m=692w/m2.
4) Summarize the output power of the PV modules and record these values, then multiply by 0.7 to get the peak value of the expected AC output.
5) Record the AC output through the inverter or system meter, and record this value.
6) Divide the AC measurement power value by the current radiation ratio, and record this value. This "AC correction value" is the rated output power of the photovoltaic system, which should be higher than 90% or more of the estimated AC value. Problems include wrong wiring, damaged fuse, inverter not working correctly, etc.
For example, a PV system consists of 20 100W PV modules, uses method 2 to estimate the solar radiation of the PV modules operating to be 692W/m2, calculates its output power at 1000W/m2, and asks the system Is it working correctly?
untie:
The total rated power of the PV array = 100 watts standard condition × 20 modules: 2000 watts normal state estimated AC output power = 2000 watts standard condition X0.7 = 1400 watts AC estimated value.
If the actual measured AC output power: 1020 watts AC measured value
Corrected AC output power = 1020 watts AC measurement ÷ 0.692 = 1474 watts AC correction
Compare the corrected AC output power value with the estimated AC output power value: 1474 watts AC fixed value + 1400 watts AC estimated value = 1.05
Answer: 1.0520.9, usually works.
This manual mainly proposes the design and installation solutions for household grid-connected photovoltaic systems. It provides installers with methods and guidelines on choosing photovoltaic products, helping them accurately install household photovoltaic power generation systems to make the design system Unleash your potential.
Ⅰ. Basic steps to follow to install a rooftop photovoltaic system
(1). Make sure the roof or other installation location is sized to accommodate the PV system that will be installed.
(2). During installation, it is necessary to check whether the roof can withstand the quality of the other photovoltaic system. If necessary, it is necessary to enhance the bearing capacity of the roof.
(3). Properly handle the roof according to the design standards of the building roof.
(4). Install the equipment strictly by the specifications and procedures.
(5). Correct and well-set grounding system can effectively avoid lightning strikes.
(6). Check if the system is running well.
(7). Ensure that the design and associated equipment can meet the grid connection needs of the local grid. 8. Finally, the system is thoroughly tested by traditional testing agencies or power departments.
Ⅱ. Problems related to system design
Types of photovoltaic power generation systems: one is a photovoltaic power generation system that is connected in parallel with the public power grid and has no backup battery for energy storage; the other is a photovoltaic power generation system that is connected in parallel with the public power grid and also has a backup battery as a supplement.
(1). Grid-connected system without battery
Such systems can only operate when the grid is available. Because the power loss of the grid is minimal, such a system can generally save the user more electricity bills. However, in a power outage, the system will shut down entirely until the grid is restored, as shown in Figure 1.
A typical battery-free grid-connected system consists of the following components:
1) Photovoltaic array.
Photovoltaic arrays consist of photovoltaic modules, which are made up of solar cells connected in some way and sealed. Usually, a collection consists of several photovoltaic modules connected by brackets.
2) Equipped with balance system (BOS)
It is used for bracket systems and wiring systems, including integrating photovoltaic modules into home building systems' electrical systems. The power supply line system includes:
- DC and AC switch at both ends of the inverter.
- Grounding protection.
- Overcurrent protection for solar cell modules.
3) DC-AC inverter
This device converts direct current from photovoltaic arrays into the standard alternating current used by household appliances.
4) Measuring instruments and meters
These instruments measure and display system operating status, performance, and user power usage. 5) Other components
Utility grid switch (this depends on the local utility grid).
(2). Grid-connected system with battery
This kind of system adds batteries to the grid-connected system without batteries to store energy for the system. Even in a power outage, the system can provide an emergency power supply for special loads. When the power is interrupted, the system is separated from the grid to form an independent power supply line. A dedicated distribution line is used to supply power to these special loads. If the power failure of the grid occurs during the day, the photovoltaic array can supply power to these loads together with the battery; if the power failure occurs at night, the battery will supply power to the load, and the battery can release enough energy to ensure the regular operation of these special loads.
In addition to all the components in a grid-connected system without a battery, a battery backup system also needs to add batteries and battery packs, battery charge controllers, and switchboards that supply power for loads with special requirements and high security.
Ⅲ. Installation of rooftop photovoltaic system
1). roof structure
The most convenient and appropriate place to install a photovoltaic array is on the roof of a building. For pitched roofs, the photovoltaic array should be installed on the roof parallel to the roof surface, with brackets separated by a few centimeters for cooling purposes. If it is a horizontal roof, it is also possible to design a bracket structure that optimizes the inclination angle and install it on the top. The roof-mounted photovoltaic system must pay attention to the sealing of the roof structure and the roof anti-permeability layer. Generally, one support bracket is required for every 100 watts of PV modules. For a new building, the support brackets are usually installed after the roof decking has been installed and before roof waterproofing has been installed. The staff responsible for the array mounting system can install the support brackets while installing the roof.
Tile roofs are often structurally designed to close their load-bearing capacity limits. In this case, the roof structure must be reinforced to withstand the additional weight of the PV system, or the tiled roof must be changed into a dedicated strip area to install PV arrays. However, if a tiled roof is converted to a lighter roofing product, there is no need to strengthen the roof structure because the combined mass of such a roof and photovoltaic array is lighter than the mass of the replaced tiled roofing product.
2). shade structure
An alternative to roof installations is a shading structure-mounted photovoltaic system. This shading structure maybe a patio or a double-layered shading grid, where the photovoltaic array becomes the shade. These shading systems can support small or large photovoltaic systems.
Such buildings with photovoltaic systems cost slightly differently than standard patio coverings, primarily when the photovoltaic array acts as a partial or complete shade roof. If the PV array is installed at a steeper angle than a typical shading structure, the roof structure will need to be modified to accommodate wind loads. The mass of the photovoltaic array is 15-25 kg/m², which is within the load-bearing limit of the shade support structure. The labor costs associated with installing roof brackets can be factored into the entire patio cover construction cost. The overall construction cost is likely to be higher than installing it on the roof, but the value generated by the shading structure often offsets those extra costs.
Other issues to consider include: simplifying the maintenance of the array, the wiring of the components, the connection of the wires must remain aesthetically pleasing, and creeping plants must not be grown or pruned to keep the members and their wiring undisturbed.
3). Building Integrated Photovoltaic (BIPV)
Another type of system replaces some traditional roofing products with building-integrated photovoltaic arrays. When installing and using such products, care must be taken to ensure that they are installed correctly, achieve the necessary fire rating, and require proper installation to avoid roof leaks.
Ⅳ.estimate the system output
1). Standard Test Conditions
Solar cell modules generate direct current. The manufacturer calibrates the DC output of the solar module under standard test conditions. While these conditions are easily achieved in the factory and allow products to differ from one another, these data need to be corrected to evaluate their output power when operating in outdoor conditions. The standard test conditions are a solar cell temperature of 25°C, a solar radiation intensity of 1000 watts/square meter (commonly referred to as peak sunlight intensity, which is equivalent to the radiation intensity at noon on a clear summer day), and a mass of 1.5 AM when passing through the atmosphere. Filtered solar spectrum (ASTM standard spectrum). Manufacturers refer to solar modules with an output of 100 watts as measured under standard test conditions as "100-watt solar modules". The rated power of this battery pack is allowed to deviate from the actual value by 4-5%. This means that a 95-watt module is still called a "100-watt module". A lower output power value should be used as a basis (95 watts instead of 100 watts).
2). temperature effect
The module's output power decreases as the module's temperature increases. For example, when the sun shines directly on the photovoltaic roof module, the internal temperature of the module will reach 50℃~75℃. For monocrystalline silicon modules, the increase in temperature will cause the module power to drop to 89% of the actual power. Therefore, a 100-watt module can only produce about 85 watts (95 watts x 0.89 = 85 watts) when it is hit by full sunlight at noon in the spring or fall.
3). Dirt and Dust Effects
The accumulation of dirt and dust on the surface of the solar panel will affect the transmission of sunlight and reduce the output power. Most areas have rainy and dry seasons. Although rainwater can effectively clean the dirt and dust on the surface of the module during the rainy season, a more complete and adequate estimation of the system should consider the power reduction caused by the dirt on the surface of the panel during the dry season. Due to dust factors, the system power is generally reduced to 93% of the original rated value every year. So this "100-watt module" runs at an average power of 79 watts (85 watts X 0.93 = 79 watts) with dust on the surface.
4). Matching and Line Loss
The maximum power output by the overall PV array is generally less than the sum of the total power output by individual PV modules. This discrepancy is caused by inconsistencies in solar PV modules, also known as module misalignment, which will cause the system to lose at least 2% of its electrical energy. In addition, electrical power will also be lost in the internal resistance of the line system, this part of the loss should be kept to a minimum. Still, it is difficult to reduce this part of the loss to the system when the power peaks at noon, and then in the afternoon Gradually decreasing again; the power will return to zero value at night; this change is attributed to the evolution of solar radiation intensity and the development of the sun angle (relative to the solar cell module). Furthermore, the inclination and orientation of the roof will affect the grade of sunlight hitting the surface of the module. The specific manifestations of these effects are shown in Table 1, indicating that if the local photovoltaic array is placed on the roof with a slope of 7:12, the correction factor facing due south is 100, when the slope angle of the roof is less than 3% of the energy. Therefore, a reasonable loss factor should be 5%.
5). DC to AC Conversion Losses
The DC power generated by the solar modules must be converted into standard AC power by an inverter. Some energy will be lost in this conversion process, and some points will be lost in the wiring from the rooftop components to the inverter and the customer switchboard. At present, the peak efficiency of inverters used in home photovoltaic power generation systems is 92% to 94%, which is the peak efficiency given by inverter manufacturers and is measured under good factory control conditions. In fact, under normal circumstances, the efficiency of the DC-AC inverter is 88%~92%, and 90% is usually used as a reasonable compromise efficiency.
Therefore, a "100-watt module" with reduced output due to product deviation, heat, wiring, AC inverter, and other power losses, at noon with a clear sky, only a maximum of 68 watts of AC power is delivered to the user's switchboard. (100WX095×0.89×0.93×095X0.90—68W).
6). Influence of sun direction angle and house orientation on system energy output
Throughout the day, the angle at which the sun's rays strike the solar panel is constantly changing, which will affect the output power. The "100-watt module" output power will gradually increase from the zero value at dawn, with the change of the sun bearing angle, the same degree. Still, the array is facing east; the power produced will be 84% of the power facing south (corrected in Table 1 factor of 0.84).
Ⅴ.System installation
1. Recommended materials
•Materials used outdoors should be resistant to sunlight and UV rays.
•Polyurethane sealants should be used on non-flash roof waterproofing. 3) Materials should be designed to withstand the temperature when exposed to the sun.
•Different metal (such as iron and aluminum) materials should be isolated from each other with insulating spacers, washers, or other methods.
•Aluminum should not be in direct contact with some materials.
•High-quality fasteners should be used (stainless steel is preferred).
•Structural member materials can also be selected: aluminum profiles, hot-dip galvanized steel, coated or painted ordinary carbon steel (only used in low-corrosion environments), stainless steel.
2. Recommended equipment and installation method
1)Make a list of all electrical equipment according to the rated voltage and rated current required in the application.
2) List the PV modules according to the relevant standards, and make sure that it has a shelf life of at least five years (20 to 25 years of life).
3) List the inverter according to the relevant standard, and make sure that it has a service life of at least five years. 4) Exposed cables and pipes should be resistant to light.
5) The system should have overcurrent protection and easy maintenance.
6) Terminals related to electricity should be tightened and fastened.
7) The manufacturer's installation instructions should install the equipment.
8) All roofs should be sealed with an approved sealant.
9) All cables, pipes, exposed conductors, and wire boxes should comply with relevant standards and regulations and ensure safety.
10) It should be ensured that the photovoltaic array is not shaded from 9:00 to 16:00 every day.
3. Matters needing attention in photovoltaic system design and installation
1) Carefully check the installation site of the photovoltaic array (such as roof, platform, and other buildings).
2) To ensure that the selected equipment is within the scope of local incentive policies.
3) Contact the local utility grid department to obtain grid connection and online test permission.
4) If it is installed on the roof when determining the installation position of the photovoltaic modules on the top, the influence of the building rainwater drainage pipes, chimneys and ventilation openings on the photovoltaic modules should be considered. Try to lay photovoltaic modules according to the size and shape of the roof to make the top more beautiful.
5) Calculate the sunlight exposure and shading of the installed photovoltaic array. If the chosen installation site has too much shade, you should consider changing where the PV array is installed.
6) Measure the distance between all system components, and draw the location diagram and schematic diagram of the photovoltaic system installation.
7) Collect relevant materials for relevant review departments, which should include the following:
①The location map shall show the location of the main system components - photovoltaic modules, pipeline wiring, electrical boxes, inverters, high-assurance load switchboards, utility grid on-off switches, main switchboards, and the inlet side of the utility grid.
②The schematic diagram should show all the essential electrical system components, as shown below picture
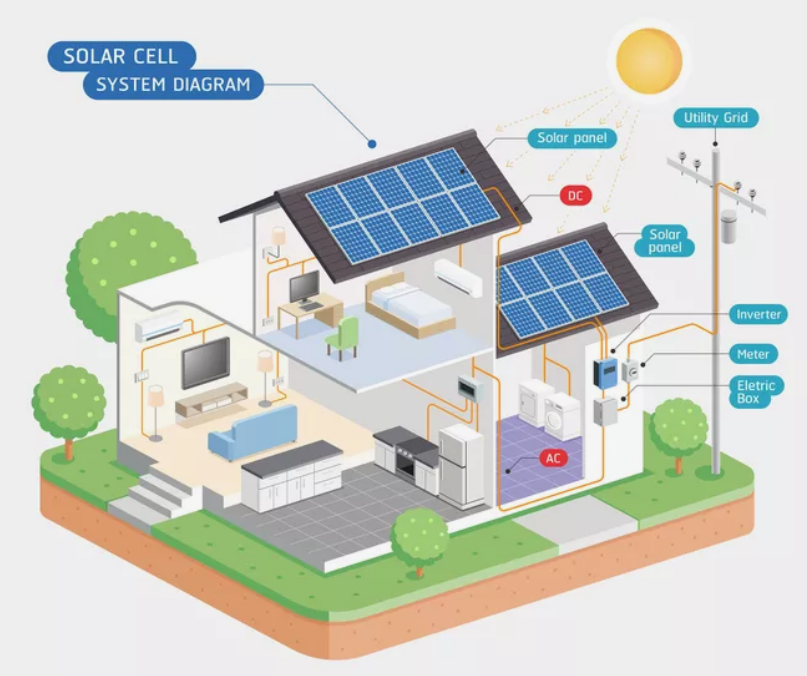
③Break down all critical electrical system components into small parts (photovoltaic modules, inverters, combiner boxes, DC switches, fuses, etc.).
8) Estimate the cable length from PV modules to combiner box and inverter
9) Check the current-carrying capacity of the photovoltaic module circuit, and determine the cable size suitable for the slightest current. The size of the cable is determined according to the maximum short-circuit current of each course and the length of the cable routing.
10) Calculate the size of the PV array, taking into account that at full power, the voltage drop from the PV module to the inverter is less than 3%. If the combiner box of the array is far from the inverter, then the voltage drop is not calculated based on the wiring from the PV array to the combiner box and the wiring from the combiner box inverter.
11) Estimate the line length from the inverter to the main switchboard.
12) Check the main switchboard to determine whether the power of the switchboard can meet the switching needs of the photovoltaic system.
13) If the system includes switchboards for support loads (with backup battery systems), identify specific critical load circuits.
These circuits should meet the expected electrical loads:
①Estimate the load connected to the backup system to meet the needs of actual power consumption and daily power consumption in the sleep state of the system.
② All backup loads must be connected to a separate switchboard for connection to the output of the dedicated inverter.
③The average power consumed by the load of the backup power system should be calculated to determine how long the energy storage in the battery can continue to supply power to the consumer.
④It is recommended to use a maintenance-free valve-regulated lead-acid battery system with adsorbed fiberglass wool because this battery does not require user maintenance.
⑤The battery's storage should avoid sunlight and be placed in a calm and ventilated place as much as possible. Whether it is a lead-acid solution or a valve-regulated lead-acid battery, it needs to be ventilated to the outside world.
14) Follow the design requirements
Cables connect PV modules, combiner boxes, overcurrent protectors/disconnect switches, inverters, and utility disconnect switches and ultimately join the circuit to the utility grid.
15) During the trial operation, the photovoltaic system circuit usually works, and the grid connection permit from the public power grid department is obtained. Then, the system can start to operate formally.
16) Observe whether the system instrument is running normally.
4. Maintenance and Operation Phase
1) When dust accumulates on the photovoltaic modules, the photovoltaic modules can be cleaned in cool weather.
2) Regularly check the photovoltaic system to ensure that the lines and brackets are in good condition.
3) Every year around March 21 and September 21, when the sun is full and close to noon, check the output of the system (the surface of the components is kept clean), and compare whether the operation of the system is close to the reading of the previous year. Keep this data in logs to analyze whether the system is always functioning correctly. If the readings drop significantly, there is a problem with the system.
Ⅵ. Inspection content and procedures of solar photovoltaic power generation system (it is recommended to wear safety helmet, gloves, and eye protection equipment)
1. PV array
1)Verify that all combiner box fuses are removed, and check that there is no voltage present at the output terminals of the combiner box.
2) Visually inspect whether any sockets and connectors between the PV modules and the switchboard are in normal working condition.
3) Check whether the stress-free clamp of the cable is installed correctly and firmly.
4) Visually inspect whether all PV modules are intact.
5) Check whether all cables are neat and fixed.
2. Circuit wiring of photovoltaic modules
1) Check the DC string combiner box (from the PV modules to the combiner box).
2) Recheck whether the fuse is taken out and all switches are disconnected.
3) Check whether the indoor cable lines are connected to the terminals of the DC series combiner box in the correct order, and make sure that the labels are visible.
3. Trace inspection of circuit string wiring
The following procedure is followed for each source circuit series in the system path (e.g., from east to west or north to south), with ideal test conditions being clear noon from March to October.
1) Check the open-circuit voltage of each component in the circuit to verify the actual voltage provided by the manufacturer on a sunny day (under the same sunlight conditions, there should be the same voltage. Note: under sunlight conditions, the have voltages above 20 volts).
2) Make sure that permanent cable markers can identify the positive and negative connections.
3) Check each component as above.
4. Other parts of photovoltaic array circuit wiring
1) Recheck that the DC disconnect switch is on and the labels are intact.
2) Verify the polarity of each branch power supply in the DC combiner box. According to the number of circuit strings and the position on the drawing, verify that the open-circuit voltage of each branch is within the appropriate range (if the sunlight irradiance does not change, the voltage should be very close).
Warning: If the polarity of any set of source circuits is reversed, this will cause a severe accident or even fire in the fuse unit, resulting in damage to the combiner box and adjacent equipment. The reversed polarity of the inverter will also cause damage to the system equipment, which is not covered by the equipment warranty.
3) Tighten all the terminals in the DC string combiner box.
4) Check that the neutral wire is connected correctly to the main switchboard.
5. Inverter startup test
1) Check the open-circuit voltage sent to the inverter DC disconnect switch to ensure that the voltage limits in the manufacturer's installation manual are met.
2) If there are multiple DC disconnect switches in the system, check the voltage at each switch.
3) Turn the power supply switch from the PV array to the inverter.
4) Confirm that the inverter is running, record the voltage of the inverter over time during operation, and confirm that the voltage reading is within limits allowed by the manufacturer's installation manual.
5) Confirm that the inverter can achieve the expected power output. 6) Provide a startup test report.
6. system acceptance test
Ideal PV system test conditions, choose sunny noonday from March to October. If ideal test conditions are not possible, this test can also be done at noon during a sunny winter day.
1) Check to ensure that the PV array is fully sunlit and without any shade.
2) If the system is not running, turn on the system running switch and let it run for 15 minutes before starting the system performance test.
3) Perform a solar irradiance test with one or two methods, and record the test value. Divide the highest radiation value by 1000 watts/square meter, and the data obtained is the radiation ratio. For example: 692w/m2÷1000w/m=0.692 or 69.2%.
Method 1: Test with a standard pyranometer or pyranometer.
Method 2: Find a normal operating photovoltaic module of the same model as the photovoltaic array, keep the same direction and angle as the photovoltaic array to be tested, and place it in the sun. After 15 minutes of exposure, use a digital multimeter to test the short-circuit current, and set the These values are recorded (in amps). Divide these values by the short-circuit current value (Isc) printed on the back of the PV module, multiply by 1000 watts/square meter, and record the results in the same row. For example: LSC measurement=36A; LSC printed on the back of PV module: 5.2A; actual radiation value=3.652A×1000w/m=692w/m2.
4) Summarize the output power of the PV modules and record these values, then multiply by 0.7 to get the peak value of the expected AC output.
5) Record the AC output through the inverter or system meter, and record this value.
6) Divide the AC measurement power value by the current radiation ratio, and record this value. This "AC correction value" is the rated output power of the photovoltaic system, which should be higher than 90% or more of the estimated AC value. Problems include wrong wiring, damaged fuse, inverter not working correctly, etc.
For example, a PV system consists of 20 100W PV modules, uses method 2 to estimate the solar radiation of the PV modules operating to be 692W/m2, calculates its output power at 1000W/m2, and asks the system Is it working correctly?
untie:
The total rated power of the PV array = 100 watts standard condition × 20 modules: 2000 watts normal state estimated AC output power = 2000 watts standard condition X0.7 = 1400 watts AC estimated value.
If the actual measured AC output power: 1020 watts AC measured value
Corrected AC output power = 1020 watts AC measurement ÷ 0.692 = 1474 watts AC correction
Compare the corrected AC output power value with the estimated AC output power value: 1474 watts AC fixed value + 1400 watts AC estimated value = 1.05
Answer: 1.0520.9, usually works.